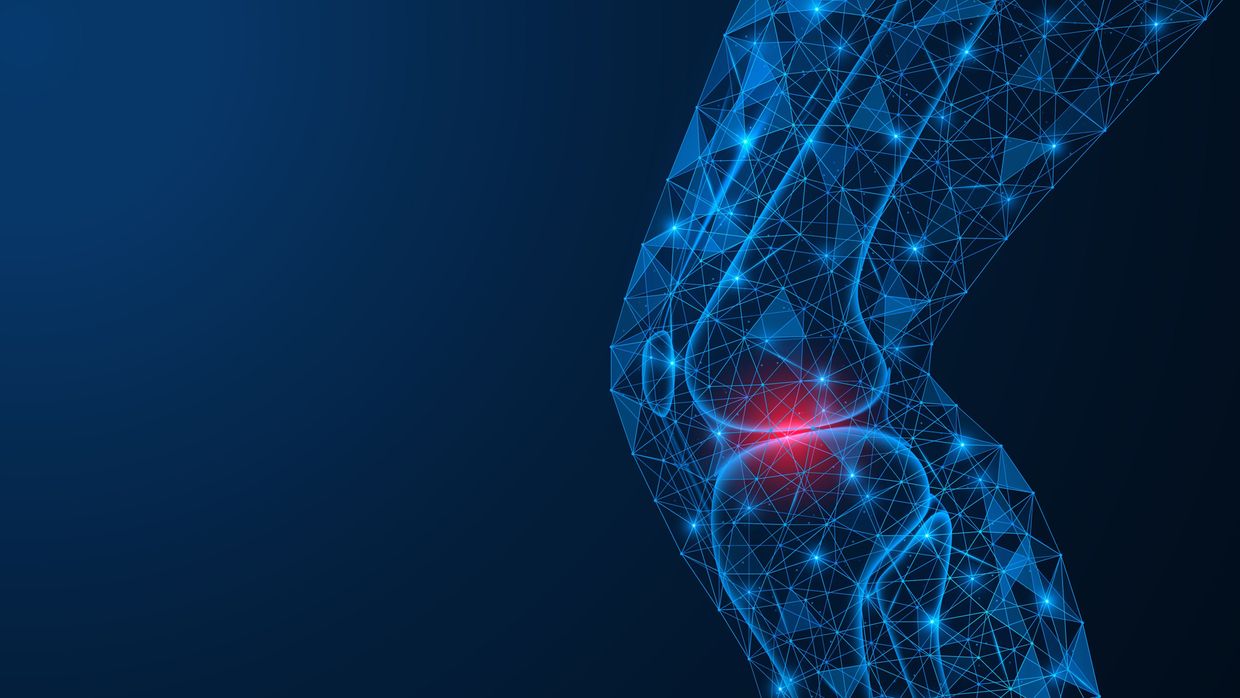
Looking for cell-based solutions to acute articular cartilage injuries
Authors:
Yann Ladner, MSc
Prof. Dr. Martin J. Stoddart
AO Research Institute Davos
Corresponding author:
Prof. Dr. Martin J. Stoddart
Cell-based therapies for articular repair have made progress, however, the lack of standardization and functional quality control results in unpredictable patient outcomes. Identifying suitable cell populations and testing them in vitro using methods that recapitulate the patients articulating joint offers hope that new therapies can be established.
Keypoints
-
Acute cartilage injury greatly increases the potential to progress to post-traumatic osteoarthritis, with the associated pain and mobility issues.
-
Marrow stimulation techniques are often employed to repair acute injury, with unsatisfactory long-term results due to the generation of mechanically inferior fibrocartilage.
-
Autologous chondrocyte implantation is an alternative cell-based therapy that requires cell culture techniques to increase cell numbers.
-
Identification of predictive functional MSC markers would improve patient stratification, thus facilitating clinical translation.
-
Recapitulating joint kinematic load in the laboratory would improve the screening of novel cell-based therapies.
Cartilage regeneration, an unmet clinical need
Acute injury to the surface of articular cartilage is notoriously difficult to treat due to the lack of an endogenous repair response. Failure to treat acute cartilage injury dramatically increases the risk of post traumatic osteoarthritis, therefore early intervention is key. However, therapeutic options for cartilage regeneration are limited. One of the most commonly applied therapies for cartilage repair is microfracture, or a more recent adaptation called nanofracture. The underlying principle of these marrow stimulation techniques, is to puncture the subchondral bone under the cartilage defect, therefore allowing mesenchymal stem cells (MSCs) contained within bone marrow into the defect. The original technique developed by Steadman utilized an awl to produce defects approximately 2–4mm deep and 3–4mm apart.8 One of the side effects of the microfracture technique is a migration of the subchondral bone into the developing cartilage tissue. This was considered to be a fracture repair response due to the damage to the underlying bone, therefore, the technique was refined to nanofracture, which uses a narrower, longer needle, with the aim to reduce the bone fracture healing response, while delivering an optimal source of cells from the deeper underlying bone.2 The infiltrating marrow then provides a source of cells that allows for repair tissue to fill the defect. Initially, this repair tissue provides pain relief and fills the defect, however, due to the de novo tissue consisting of type 1 fibrocartilage with little collagen type 2, the mechanical resilience is not maintained, and the tissue will breakdown overtime. It should be remembered that due to the infill of adult endogenous MSCs, this is actually a cell-based therapy even though no cells are directly implanted. Early studies in rabbits with this method indicated that the main source of reparative cells within the defects do indeed come from the bone marrow. However, whether this is the case in patients or in larger animals has never fully been clarified.
Cell-based therapies for cartilage repair
Due to the unsatisfactory results obtained with microfracture, other therapies are being explored. Autologous chondrocyte implantation (ACI) is a clinically approved therapy whereby chondrocytes are taken from a non-load bearing area of the patient’s joint.3 The harvested tissue is sent to a cell culture lab, where the cartilage tissue is digested, the cells or chondrocytes are then harvested from the tissue, and these cells are expanded in monolayer to obtain a sufficient number. Expanded chondrocytes in the first-generation ACI therapy were injected into the cartilage defect under a periosteal flap. However, newer iterations of ACI have the cells seeded on a membrane or matrix scaffold, which holds the cells in place and eliminates the need for a periosteal flap. This reduces one of the earliest complications with first generation ACI, which was periosteal hypertrophy that would require a second corrective surgery. Other issues remain, such as the dedifferentiation of the chondrocytes during the expansion phase.
In developing new cell-based therapies, many groups including our own have investigated the use of bone marrow-derived autologous mesenchymal stem or stromal cells (MSCs). This requires a bone marrow sample to be harvested, the cells contained within to be plated onto tissue culture plastic and, after a period of cell expansion, significant numbers of cells can be obtained that have multipotent capacity. In the laboratory, it has been demonstrated these cells can differentiate into chondrocytes (cartilage), osteocytes (bone) and adipocytes (fat), and it is this property which has led to their proposed use in cell-based therapies for cartilage. Classical in vitro based studies direct the cells towards chondrogenesis using exogenous transforming growth factor beta protein (TGFß). While this method shows promise during in vitro studies, clinical transition has yet to be realized. In part, this is due to a lack of stable robust chondrogenic differentiation. During in vitro studies, differentiation towards a chondrocyte has the tendency to result in an unstable cartilaginous phenotype that expresses typical markers of hypertrophy, such as collagen X, indicating an endochondral ossification pathway, similar to secondary bone fracture healing. How this relates to the classical microfracture complication of type 1/2 fibrocartilage is unclear, but it suggests the expanded MSCs being used for in vitro studies and tissue engineering have different properties to the naive cells migrating into a defect during marrow stimulation therapies.
Predicting monolayer expanded cell function is challenging
When considering monolayer expanded cells, one major challenge is that despite the use of reproducible and robust protocols during cell preparation, functional variation between donors can be significant. The outcome from a functional tissue perspective often varies, and this variation cannot be predicted in advance. Typically, when using MSCs as a cell source, cluster of differentiation (CD) markers are employed to define and characterize the cells as MSCs. While this provides a rudimentary characterization and can distinguish the mesenchymal cells from hematopoietic cells, the use of CD markers to date has not correlated with any functional cell outcome. When considering the need for a clinically applicable, cell-based therapy, there will be a need to characterize the cells before their release and implantation into the patient. This would bring cell-based therapies into line with common practices for pharmaceutical products, thus enabling a greater uptake in use. Therefore groups, including our own, are investigating the potential to find functional markers of MSC biology that will predict the chondrogenic potential of a specific cell population. One of the first markers was established by the group of Hollander where the retinoic acid receptor-related orphan receptor (ROR) was shown to correlate to MSC chondrogenesis.4 We also discovered the ratio of TGFß receptor 1/receptor 2 to be a robust marker of chondrogenic potential.6 An advantage of this marker is that it can easily be investigated using standard polymerase chain reaction methods (PCR). Furthermore, the receptor profile on the day the cells are harvested from tissue culture plastic is predictive of the chondrogenic potential of the cells after 28 days in vitro culture. To date, this marker has been demonstrated to be effective for bone marrow MSCs, however it remains to be seen if this predictive efficacy is valid when using MSCs derived from different tissues such as adipose. When considering donor stratification, it would be advantageous if the marker established not only identified poor responsive donors but could also be used as a target, therefore allowing non-responsive donors to be treated in a way that allowed them to still have clinical use. As the profile identified is a ratio of two TGFß receptors it is feasible that correcting the ratio would then reverse the deficit in chondrogenic potential. Indeed, we demonstrated that reversing the poor receptor ratio from a non-responsive donor with a simple application of siRNA or TGFß protein led to a reversal of the non-responsive phenotype and recovered the chondrogenic potential of poor donors.1,6 This would offer a standardized route by which monolayer expanded MSCs could be primed for chondrogenesis and therefore increase the reproducibility of implanted cells.
When implanting MSCs into an articular defect there is still the question as to whether cell differentiation, or the cell secretome, is the main mechanism of action. While cell differentiation has been studied for many years, recently, interest is growing in the secretome produced by the cells. The cell secretome consists of growth factors and proteins, but cells can also produce extracellular vesicles that contain messenger and noncoding RNA that allows for cell-to-cell communication. The exact mechanism of action may also be dependent on the cell culture methods used to obtain the implanted cell population.
Testing cell-based therapies in the lab under the correct mechanical environment
One additional complication of translating cell-based therapies into the clinic is the design of the testing platform itself. Typically, chondrogenesis is induced in static cell culture models in a 3D environment using exogenous TGFß protein. It is clear from studies involving patients that cells of the musculoskeletal system, including cartilage, are mechanoresponsive. The classical static cell culture models therefore do not recapitulate the mechanical forces the cells would be exposed to when applying rehabilitation protocols during healing. Therefore, our group employs complex multiaxial load bioreactor systems that can apply a combination of compression and shear, mimicking the kinematic loads typically applied in articulating joints. Using this system, we have been able to induce chondrogenic differentiation of human bone marrow-derived MSCs using mechanical forces alone.7 We identified that a combination of compression and shear is successfully able to mechanically activate the latent TGFß protein produced by the cells themselves, therefore allowing a chondrogenic differentiation to take place.5 This provides insights into potential new rehabilitation protocols and suggests that early rehabilitation should be applied after cell-based therapies for articular cartilage repair when considering cell differentiation as a primary outcome. Indeed, Steadman recommends that continuous passive motion (CPM) is begun in the recovery room immediately after surgery, emphasizing the importance of early mobilization. We are further developing these complex models to incorporate aspects such as bone and cartilage by implementing an osteochondral plug defect, which can then be stimulated with kinematic joint mimicking articular load. The next step in this development is to transfer the whole loading bioreactor system into physioxia conditions, whereby the culture system will be maintained from start to finish under physiological oxygen that would typically be found in the joint environment, which is around 2%. Classical in vitro studies are performed in controlled cell culture incubators at atmospheric oxygen which is approximately 20%. This is clearly too high when considering the patient, and it is expected that lowering the oxygen content to 2% will lead to more clinically translatable results. Thus, the ultimate model will contain cartilage, bone, mechanical load and the correct oxygen tension, thereby providing a more accurate representation of the patient’s articulating joint. This should lead to improved testing of novel therapies in vitro. This will reduce the use of animals during the testing phase, as it will be possible to better predict potential therapeutic value when prescreening therapies prior to implantation into a large animal model.
Opportunities to collaborate
To obtain clinically relevant data, we exclusively use human derived material such as bone marrow and periost. In this regard, we are always looking for clinical collaborators. If you would like to get involved, please contact Prof. Martin Stoddart to discuss possibilities.
Acknowledgements of funding sources:
This work has been funded by the AO Foundation, Swiss National Funds grants 31003A_179438/1 and 31003a_146375/1, and EU H2020 OSTASKILLS H2020-MSCA-COFUND-2020 and CARTHAGO H2020 ITN Marie Skłodowska-Curie grant agreement No 955335.
Literature:
1 Basoli V et al.: Effect of expansion media and fibronectin coating on growth and chondrogenic differentiation of human bone marrow-derived mesenchymal stromal cells. Sci Rep 2021; 11(1): 13089 2 Behrens P et al.: Reviewing subchondral cartilage surgery: considerations for standardised and outcome predictable cartilage remodelling: a technical note. Int Orthop 2013; 37(11): 2139-45 3 Brittberg M et al.: Treatment of deep cartilage defects in the knee with autologous chondrocyte transplantation. N Engl J Med 1994; 331(14): 889-95 4 Dickinson SC et al.: The wnt5a receptor, receptor tyrosine kinase-like orphan receptor 2, is a predictive cell surface marker of human mesenchymal stem cells with an enhanced capacity for chondrogenic differentiation. Stem Cells 2017; 35(11): 2280-91 5 Gardner OFW et al.: Joint mimicking mechanical load activates TGFbeta1 in fibrin-poly(ester-urethane) scaffolds seeded with mesenchymal stem cells. J Tissue Eng Regen Med 2017; 11(9): 2663-6 6 Rothweiler R et al.: Predicting and promoting human bone marrow MSC chondrogenesis by way of TGFbeta receptor profiles: toward personalized medicine. Front Bioeng Biotechnol 2020; 8: 618 7 Schatti O et al.: A combination of shear and dynamic compression leads to mechanically induced chondrogenesis of human mesenchymal stem cells. Eur Cell Mater 2011; 22: 214-25 8 Steadman JR et al.: Microfracture: surgical technique and rehabilitation to treat chondral defects. Clin Orthop Relat Res 2001; (391 Suppl): S362-9
Das könnte Sie auch interessieren:
Gesundheitskosten steigen weniger stark, Löhne aber auch
In die Debatte über die Initiativen zu den Gesundheitskosten platzen nun neue Zahlen zum Anstieg der Kosten. Dieser fiel weniger stark aus. Allerdings sind die Reallöhne sogar leicht ...
Debatte über neue Arzneimittel geht weiter
Grosse Sorge um den Zugang zu neuen Medikamenten: Die ständerätliche Gesundheitskommission (SGK-S) will Preissenkungen für Medikamente mit hohen Umsätzen.
Universitätsspital baut Digitalisierung aus und setzt auf KI
Das Universitäts-Kinderspital beider Basel (UKBB) intensiviert seine Zusammenarbeit mit Microsoft und nutzt die Technologien, um Abläufe im Spital zunehmend zu automatisieren.